How It's Done: Photosynthesis
- Aarushi Gupta
- Nov 21, 2019
- 7 min read
Updated: May 23, 2020
For a Biology assignment, we had to make a study artifact and we had to be creative with it. This post will be my creative component. This post will also be a glimpse of what I really want this blog to be. Since I made handwritten notes for the first half of this unit, Metabolic Processes, I decided to make a blog post explaining the second half, Photosynthesis.
Here is a brief, yet not so brief, explanation of photosynthesis.
6Co2+6H2O ->C6H12O6+6O2
If you've taken a science course at any point in your life after grade 5, this equation will look familiar to you. It's the overall, condensed way of showing what happens in photosynthesis. But, in reality, photosynthesis isn't just one step.
Photosynthesis occurs in organisms, photoautotrophs, that harness light to make food; they convert light energy into usable, chemical energy. They take in the light energy released by the Sun and CO2 in the atmosphere and make glucose molecules that can be used in cellular respiration or be stored in the form of starch. These organisms still carry out cellular respiration to get energy for other processes, but they require photosynthesis to make the glucose needed for cellular respiration.
These organisms have specialized organelles to carry out the processes. For example, in plants, leaves are the site of photosynthesis; more specifically, organelles called chloroplasts. Chloroplasts are double membraned (like mitochondria) organelles, containing stacks of coin-like structures, thylakoids. You can think of them as tiny, little pancakes, stacked one on top of the other; the stack is called a granum. Pigments like chlorophyll and carotenoids are found in the membrane of the thylakoids. They are placed in such a way that no matter where the light hits the leaf, it can be absorbed by the pigments.
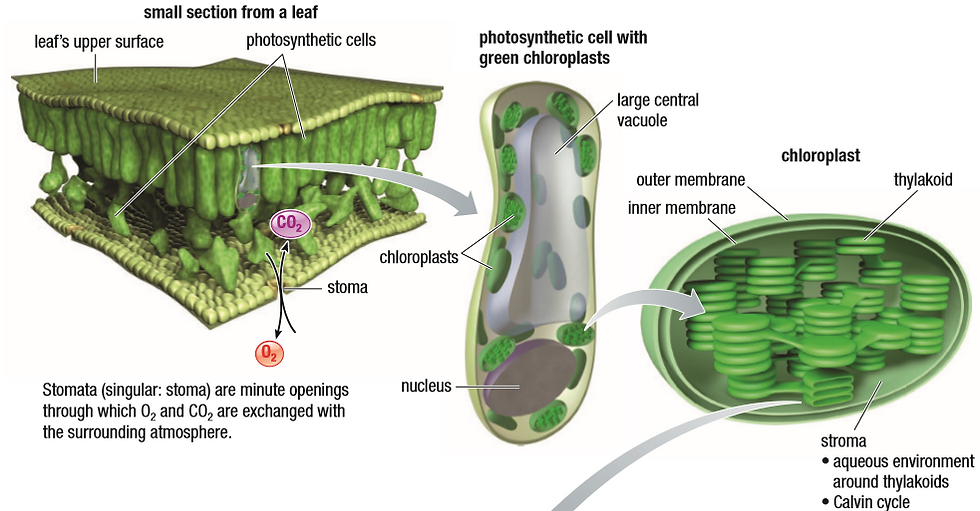
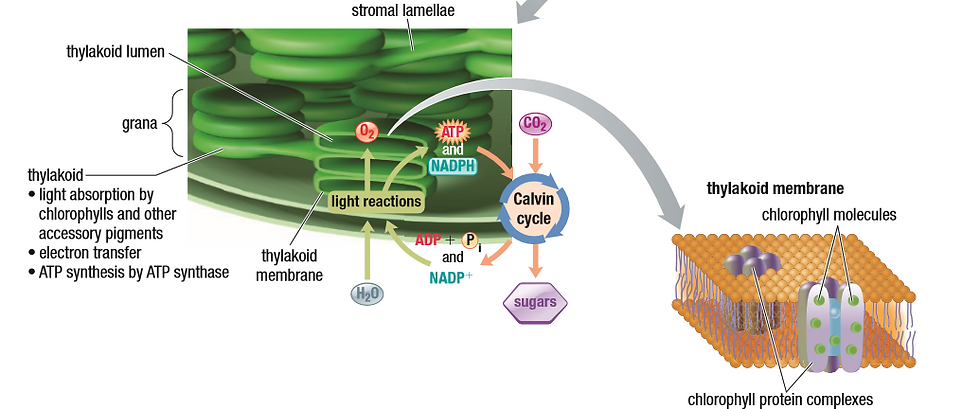
*The reason why leaves generally look green is that chlorophyll a & b and carotenoids absorb red, orange, blue and purple light. That is the wavelength that allows them to do photosynthesis. They reflect the unused green and a little bit of yellow light that reaches our eyes. In the fall, the temperature drops below the optimal temperature needed for chlorophyll a and b to function. They stop absorbing red, orange, blue and purple light. The carotenoids only absorb blue and purple light, reflecting the rest of the colours. Since red and orange light have a higher wavelength than green light, they reach our eyes first and we believe the leaves have turned red, orange and yellow.*
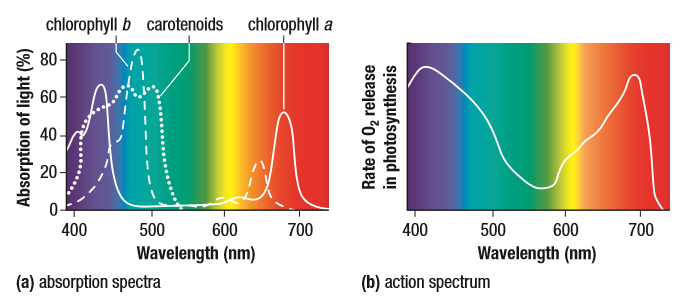

There are 2 important processes of Photosynthesis:
1. Light-Dependent Reactions
2. Light-Independent Reactions (Calvin Cycle)
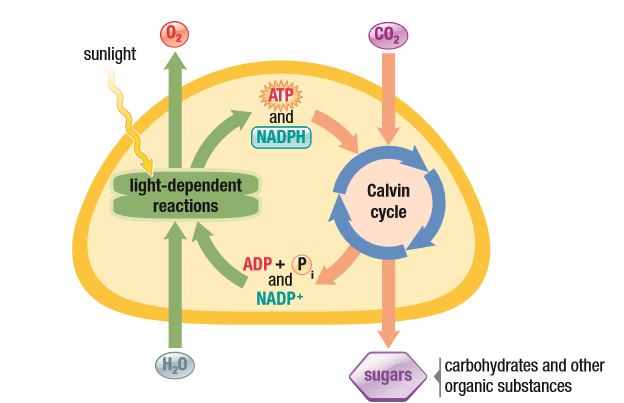
1. Light Dependent Reactions:
These reactions begin when a photon of light strikes a photosystem in a chloroplast. A photosystem is a chlorophyll molecule and its accessory/antenna pigments embedded in proteins that are a part of the thylakoid membrane.
The first part of these reactions is Photoexcitation:

The photon strikes an electron of chlorophyll a (aka the reaction centre), which absorbs the energy, gets excited and jumps to a higher state. There are plenty of accessory pigments that make sure that the energy of the photon reaches the chlorophyll, even when the light doesn’t directly hit it.
Due to the instability of the electron, a primary electron acceptor (pheophytin) captures it. Without the acceptor, the electron would drop back to the chlorophyll. Since that would be a waste of energy, the acceptor carries the electron away to the next process. This oxidizes the chlorophyll molecule and reduces the acceptor.
The next part of the reactions is the Non-Cyclic Electron Flow or Electron Transport Chain. This part is similar to the ETC of cellular respiration.
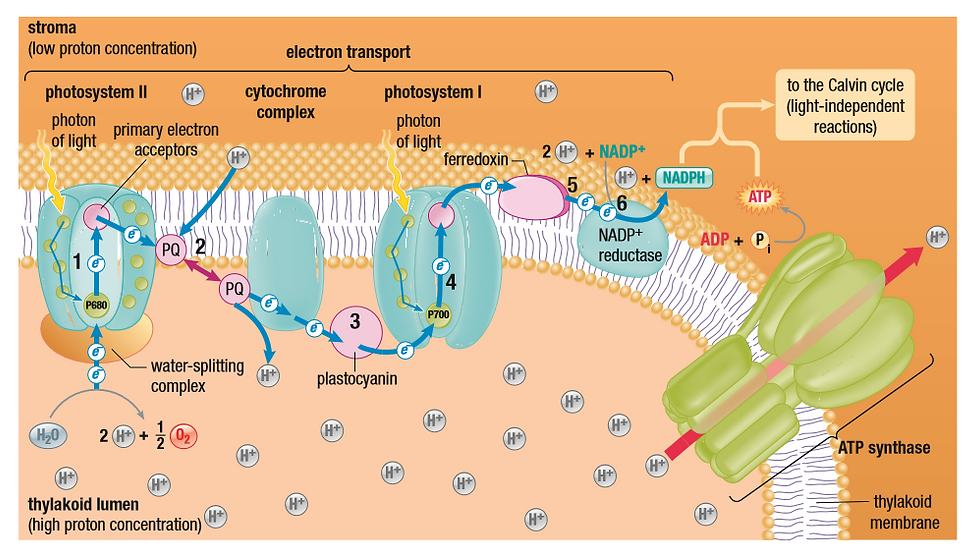
There are two photosystems involved in this process, Photosystem I and II. The difference is that in the photosystem that comes first, Photosystem II, chlorophyll-a absorbs light most at a 680nm wavelength (red light) and is known as P680. In the next one, Photosystem I, chlorophyll absorbs light mostly at a 700nm wavelength (still red light). This difference is only because of the proteins they are associated with.
Like the ETC of cell respiration, the transfer of electrons creates enough energy to pump H+ ions from the stroma (the space inside the chloroplast) of the thylakoid into the lumen (the space inside the thylakoid) of the chloroplast.
This energy comes from the movement of the electrons, from a water molecule, which is split with the help of a Z protein, to photosystem II to pheophytin to a mobile electron carrier, plastoquinone, to the cytochrome b6-f complex. The b6-f complex acts as a proton pump (a proton here is a hydrogen ion because an H+ ion has lost its electron and only has a proton left). The pump allows 4 H+ to enter the thylakoid. This contributes to making a concentration gradient of the H+ for later use.
After the b6-f complex comes photosystem I. Here the same process of photoexcitation happens. An electron is excited and is taken by an acceptor. This electron is replaced by the electron that travelled from photosystem II. Photosystem I is oxidized and the mobile electron carrier, ferredoxin, is reduced. It takes the electron to the enzyme, NADP reductase. The name is self-explanatory; it reduces NADP+ to NADPH. This will be useful in the light-independent reactions. Since 2 electrons are needed to reduce NADP+ to NADPH, this set of reactions happens twice. The images show the movement of 2 electrons at once only for the convenience of not having to draw it twice.
The last part of the light-dependent reactions is Chemiosmosis:
The protons gather in the lumen and, with the help of the electrochemical gradient formed, pass through ATP synthase, an enzyme that phosphorylates ADP to ATP, to the stroma. The H+ ions are reused in the reactions again and the 1 ATP made for every 4 H+ ions go to the next set of reactions. This called photophosphorylation because ATP synthesis would not happen without any light hitting the chlorophyll.
In some cases, there can be a cyclic flow of electrons, where the ATP synthase isn't needed to synthesize ATP. This only happens in photosystem I where the electron lost is carried by ferredoxin to plastoquinone and back to photosystem I. This makes ATP but no NADPH to go to the next set of reactions, without which the characteristic carbon fixation cannot happen.
*The reason why plants breathe in oxygen and release carbon dioxide at night is because there is no sunlight to excite electrons that will make ATP and NADPH for the next set of reactions. At night, they switch to doing just cellular respiration in the cytoplasm and mitochondria, which is why they take in O2 and release CO2 as a waste product.*
2. Light Independent Reactions - The Calvin Cycle
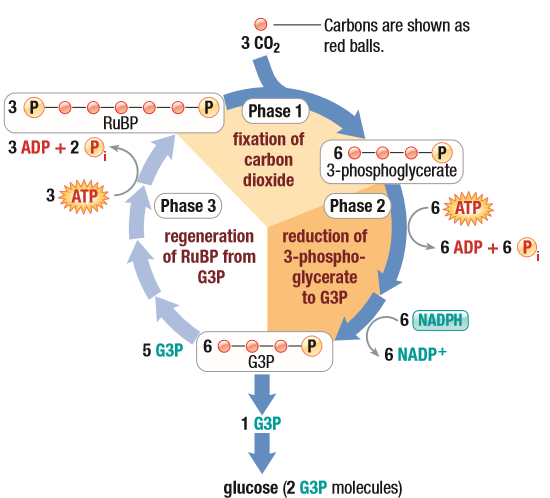
This set of reactions is similar to the Krebs cycle. It converts the CO2 harnessed from the air into usable energy in the form of glucose. Here, the reactions have moved from the lumen of the thylakoid to the stroma and as the name suggests, it's a cycle, where the first reactant is also the final product.
The first two phases of these reactions are Carbon Fixation and Reduction Reactions:
This part will be a little confusing but I wish I knew a better way to explain it. A glucose molecule has 6 Carbon atoms. One cycle makes a 3 Carbon molecule, glyceraldehyde- 3-phosphate (hereon, G3P). To get to the 6 Carbons, the cycle happens twice. To get to the 3 Carbons, 3 Carbon Dioxide molecules are used, one at a time. But to save this post from being too long, we'll assume it happens all at once.
3 5-Carbon molecules, ribulose-1,5-bisphosphates (hereon, RuBP), are found in the stroma of the chloroplast, waiting for 3 Carbon atom. 3 water molecules come and oxidize the 3 CO2 molecules to give the 3 RuBPs the 3 Cs. With the help of a large and slow enzyme, rubisco, 3 unstable, 6-Carbon molecules are made that instantly split into a total of 6 3-Carbon molecules, 3-phosphoglycerate (PGA). 6 ATP molecules, one for each PGA, come and lose a phosphate to the PGA. This makes 6 1,3- bisphosphoglycerates (1,3 BPG). Next, the NADPH molecules that were made in the previous reactions come and reduce the 1,3 BGP and become oxidized NADP+. This allows one of the 2 phosphate groups to leave the molecule. This makes 6 G3P molecules. Interestingly, only 1 G3P molecule leaves the cycle. Now, there are 5 3-C molecules left.
The last phase of the Calvin Cycle is the Regeneration of RuBP:
There are several steps of rearrangement of the 5 3-C molecules to make the 3 5-C molecules that will be ready to accept the next 3 CO2 molecules. In these rearrangements, 2 molecules of water come and reduce the 3-Cs to allow 2 phosphates to leave. 3 ATPs are also used. You start with 5 3-Carbon molecules and end with 3 5- Carbon molecules. SO, the number of Carbons remains constant.
In total, 1 Calvin Cycle uses 9 ATP and 6 NADPH molecules, which are provided by the light-dependent reactions, so these reactions are not light-independent after all. The cycle must happen twice to get 2 G3Ps to make one molecule of glucose, so, 18 ATP and 12 NADPH are used.
The glucose is then used for a variety of processes:
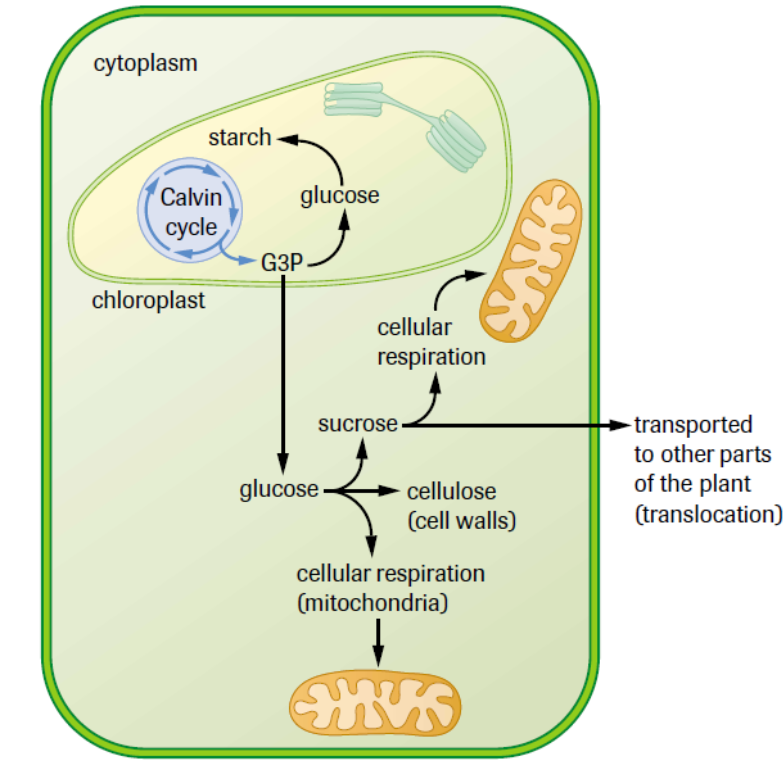
Cellular respiration: If you look at the chemical equations of cell respiration and photosynthesis, you will notice that the products of one are the reactants of the other. So, the glucose made from the Calvin Cycles can go straight to cell respiration to be broken down for energy.
Sucrose formation: Sucrose is the easiest to transport through the plant so the glucose made can be converted to sucrose and be sent to parts that need it. The sucrose can then go to cell respiration and be broken down in usable glucose and fructose.
Starch formation: The plant may not need to break glucose down for extra energy, so it is converted into the polysaccharide starch. Starch is stored as granules in and around the chloroplast, waiting to be used.
There are plenty of similarities and differences between cell respiration and photosynthesis so here is a comparison of the two:
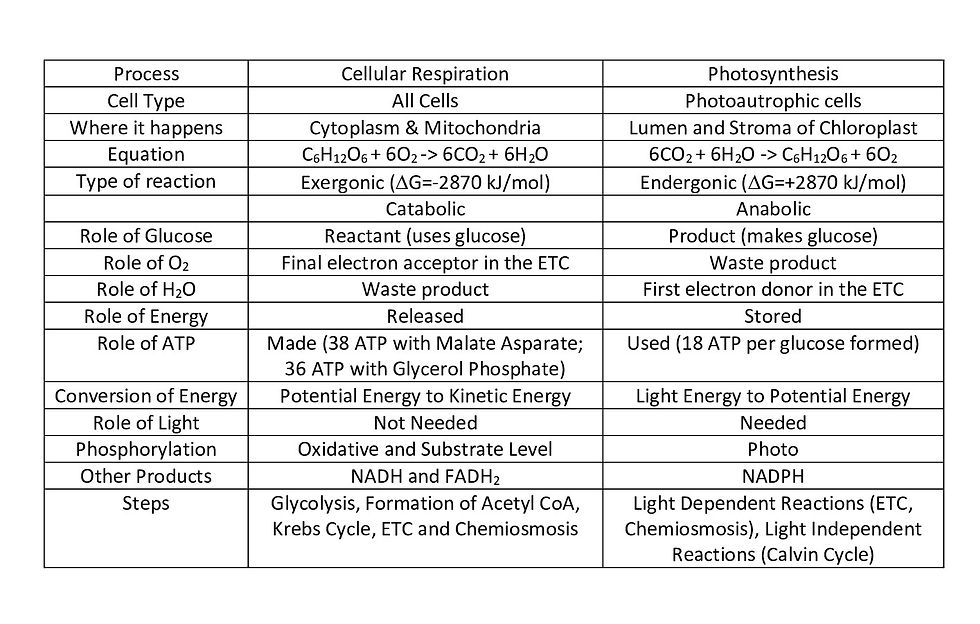
If someone had told me 5 months ago, that I would be explaining photosynthesis through a blog post, I would have said that was impossible, because a) I don't have a blog to do that and b) I don't like the Biology of plants. But, oh how the tables have turned. Now, not only do I like plants, I understand what most of what I've been learning about plants actually means. Why is water a waste product in cell respiration and a reactant in photosynthesis? Why do plants breathe in oxygen at night and release carbon dioxide? Thanks to my teacher, I get the importance of knowing all of this. You rock, Ms. Jackman!
I really hope this was a helpful post. Let me know if you'd like to see more. I might do one later in this semester, so stay tuned for that one. - Aarushi
APA Citation:
Fraser, D., LeDrew, B., Vavitsas, A., White-McMahon, M. (2012). Biology 12. Toronto: Nelson Education Ltd.
Comentarios